'Observations show that Antarctic ice shelves gained 661 Gt of ice mass over the past decade'
More evidence from the peer-reviewed literature suggests 'observations' (science) trumps (pun intended) 'estimates' ('the Science™'), courtesy of Andreasen et al. (2022), in The Cryosphere
This is another instalment of the occasional series ‘Climate Change Watch’. Please find the first two parts—one about Norwegian mountain passes having been ice-free some 1500 years ago and the other about ice-free summits in the Alps c. 3,350 years ago—in these pages.
Today, I would like to direct your attention to yet more evidence about ambiguities in mainstream assumptions and potential flaws in climate modelling used to increase the fear factor about sudden doom.
I would like to discuss the following paper today: Andreasen, J. R., Hogg, A. E., and Selley, H. L.: ‘Change in Antarctic ice shelf area from 2009 to 2019’, The Cryosphere, 17, 2059–2072, https://doi.org/10.5194/tc-17-2059-2023, 2023.
Changes in Antarctic Ice Shelf Area, 2009-19
In their abstract, Andreasen et al. write (emphases mine):
Antarctic ice shelves provide buttressing support to the ice sheet, stabilising the flow of grounded ice and its contribution to global sea levels. Over the past 50 years, satellite observations have shown ice shelves collapse, thin, and retreat; however, there are few measurements of the Antarctic-wide change in ice shelf area. Here, we use MODIS (Moderate Resolution Imaging Spectroradiometer) satellite data to measure the change in ice shelf calving front position and area on 34 ice shelves in Antarctica from 2009 to 2019. Over the last decade, a reduction in the area on the Antarctic Peninsula (6693 km2) and West Antarctica (5563 km2) has been outweighed by area growth in East Antarctica (3532 km2) and the large Ross and Ronne–Filchner ice shelves (14 028 km2)…Overall, the Antarctic ice shelf area has grown by 5305 km2 since 2009, with 18 ice shelves retreating and 16 larger shelves growing in area. Our observations show that Antarctic ice shelves gained 661 Gt of ice mass over the past decade…
That final sentence is cut off—for one reason: it shows the beauty and elegance of ‘The Scientific Method’ in action (and I don’t want to highlight all of it but for the few key words):
Our observations show that Antarctic ice shelves gained 661 Gt of ice mass over the past decade, whereas the steady-state approach would estimate substantial ice loss over the same period, demonstrating the importance of using time-variable calving flux observations to measure change.
In this one admittedly slightly convoluted sentence, Andreasen et al. take a sledgehammer and deal a significant amount of damage to ‘models’. In other words: their ‘old-fashioned’ observations show something that estimates deriving from models could not.
On Methodological Problems in Modelling
From the above-related piece, with my emphases added:
Over the past 30 years, ice shelves across Antarctica have been observed to advance steadily, retreat after iceberg calving events, and collapse catastrophically, as seen in the case of the Larsen A (Rott et al., 1996), Larsen B (Rack and Rott, 2004), and Wilkins ice shelves (Padman et al., 2012) on the Antarctic Peninsula. Tracking the change in the calving front location is a vital input parameter for ice flow models, as it is used to inform studies of calving processes and their driving forces (Trevers et al., 2019) and is required to compute ice shelf mass change from calving, a component part of the total budget along with basal melt and surface mass input (Rignot et al., 2013). Measurements of the ice shelf calving front location have been made using a range of methods, including historical ship-based observations dating from 1842 on the Ross Ice Shelf (Jacobs et al., 1986; Keys et al., 1998), manual delineation of images acquired by aerial photography (Cook et al., 2005), and optical and synthetic aperture radar (SAR) satellites (Cook and Vaughan, 2010; MacGregor et al., 2012), automated ice front detection (Baumhoer et al., 2019), and by applying edge detection techniques to satellite radar altimetry elevation data (Wuite et al., 2019). The spatial resolution, accuracy, and frequency of these complementary techniques vary, with the temporal and spatial extent of calving front measurements largely dependent on the repeat period and coverage of data acquired and the manual intensity of the processing technique used. While data prior to the satellite era (pre-1960s) are extremely limited, historical records are an important reference dataset for understanding long-term change in the ice front position and its response to environmental forcing…In this study, we expand on this previous work and provide a circum-Antarctic survey by mapping the annual calving front location on 34 ice shelves around Antarctica from 2009 to 2019, using MODIS (Moderate Resolution Imaging Spectroradiometer) satellite imagery (Scambos et al., 1996). The results provide a comprehensive assessment of ice front migration across Antarctica over the last decade, expanding on historic patterns of ice movement and enabling areas of growth and retreat to be accurately quantified (Fig. 1).
So, basically, data before the 1960s is problematic, which reduces the accuracy of ‘models’ and ‘reconstructions’ based on ‘historical records’ and a range of proxies used, such as ice-core samples etc.
This is further explained in the methods section of the paper:
We measured the annual calving front position on 34 ice shelves, encompassing 80 % of the Antarctic coastline, over the 11 years from 2009 to 2019 (Fig. 1). We used over 350 multispectral optical images, acquired by the MODIS instrument on board the NASA Terra and Aqua satellites (Scambos et al., 1996; Table S1 in the Supplement)…The study period started in 2009 on 30 ice shelves; however, on the Wordie, Baudouin, Nansen, and Drygalski ice shelves, suitable images were not acquired until 2011. Therefore, this year was used as the earliest start date in these three regions throughout this study (Table 1). We produced an annual measurement of the ice front position on 34 ice shelves around Antarctica by manually delineating the calving front location at the point where the ice shelf surface visibly transitioned to open ocean or sea ice in each satellite image (Cook et al., 2005; Cook and Vaughan, 2010).
See: no reliable and/or useful data inputs for parts of Antarctica (collected via this method) before 2009-11. Here’s fig. 1 showing a visualisation of the findings:
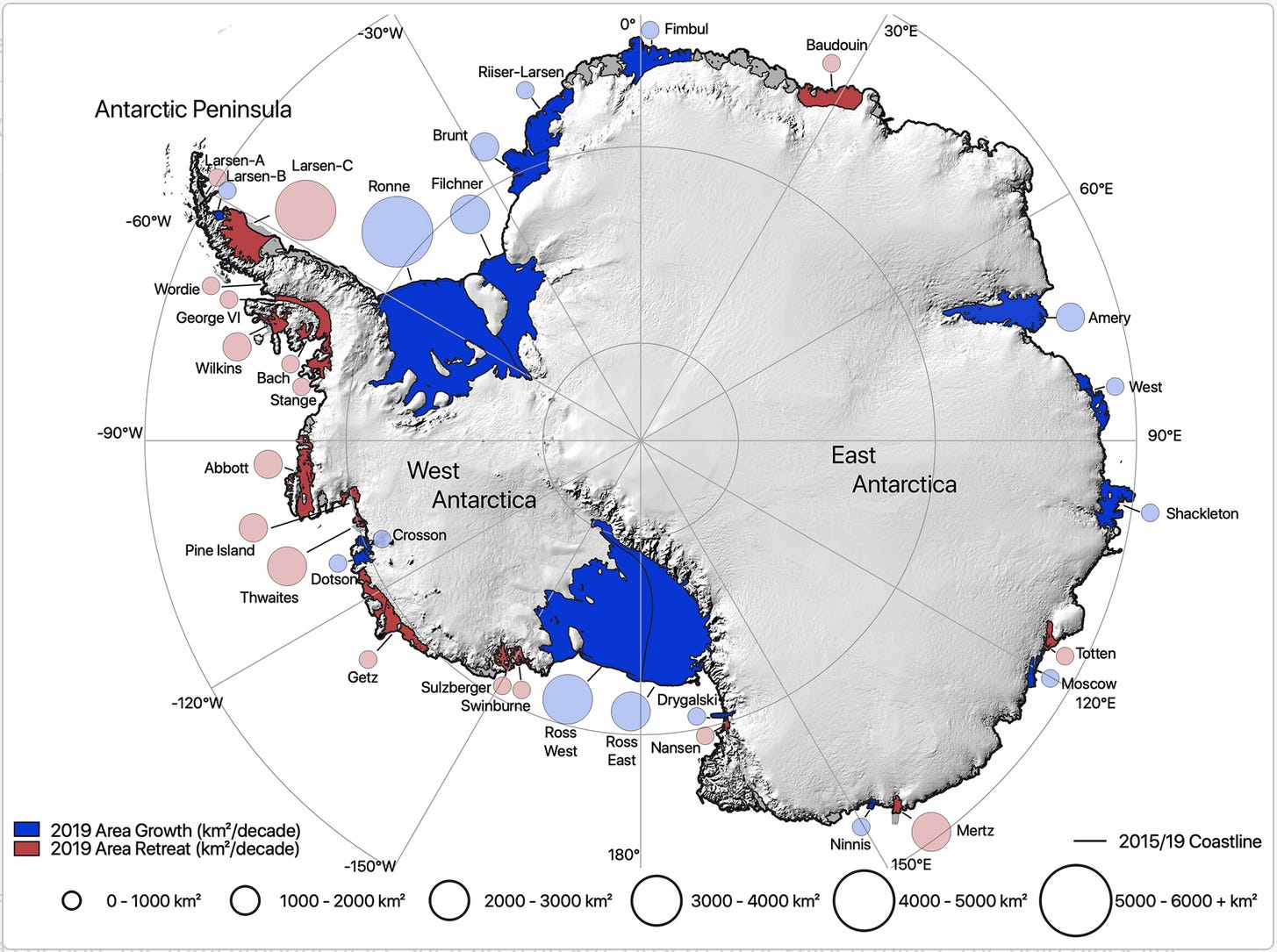
Results and Discussion
This study presents a spatially and temporally extensive record of the calving front location and area change for 34 major ice shelves in Antarctica, from 2009 to 2019 (Fig. 1; Table 1), with three of those shelves measured from 2009 to 2021. Over the 11 years from 2009 to 2019, we observed six distinct types of ice shelf calving front behaviour characterised by (a) major calving events, (b) rapid calving front retreat, (c) gradual calving front retreat, (d) advance with periodic retreat, (e) rapid calving front advance, and (f) steady calving front advance (Fig. S1 in the Supplement). We grouped the ice shelves into these six categories to describe the changes observed to provide a detailed evaluation of ice shelf behaviour in Antarctica over the last decade.
There is an extensive discussion of these six types of calving events, which I’ll spare you here; do venture over to linked article and read it.
Overall Changes
Our results show that, over the 11 years from 2009 to 2019, ice shelves in Antarctica gained a modest 0.4 % (or 5305 km2) of their total ice area (Table 1; Fig. 1). This area gain was dominated by significant 14 028 km2 (1.5 %) ice shelf area gains on the two largest Antarctic ice shelves, Ronne–Filchner and Ross, and a 3532 km2 (1.3 %) area gain on the East Antarctic ice shelves. This counteracted the large reduction in ice shelf area on the Antarctic Peninsula, where 7.0 % (−6692.5 km2) of ice was lost, and West Antarctica, where ice shelves lost 5.5 % (−5563 km2) of their 2009 area. From 2009 to 2019, our observations show that the WAIS and Antarctic Peninsula (AP) experienced overall cumulative mass loss, whereas the AP, Ross, and Ronne–Filchner saw cumulative ice mass growth (Fig. 4). Ice shelves along the West Antarctic Ice Sheet lost 150.2 Gt yr−1 of ice mass, with individual drainage basins including Pine Island, Thwaites, and Abbot contributing the most ice loss. On the Antarctic Peninsula, ice shelves also lost a total mass of 104 Gt yr−1 over the last decade, contributing significantly to freshwater input into the ocean. Larger shelves such as Ross, Ronne, and Filchner, gained 262 Gt yr−1 of ice. In East Antarctica, Baudouin, Totten, Mertz, and Nansen were the only shelves to lose ice (−5, −4, −50, and −2 Gt yr−1, respectively); however, the region as a whole gained 51 Gt yr−1 of ice from 2009 to 2019.
Steady-State Calving Flux
In the absence of an observed measurement of ice loss from iceberg calving, previous studies have used the steady-state calving approximation to estimate the volume of ice lost through calving processes (Rignot et al., 2013; Depoorter et al., 2013). This method assumes that all ice flow through a fixed flux gate, usually located near or at the last known calving front position, is lost through iceberg calving (Rignot et al., 2013). We calculated the mass change from both the observed and steady-state calving flux methods for all 34 ice shelves in Antarctica to assess the impact of fully accounting for observed change over the last decade (Table 1). We calculated the ice mass change using the observed calving flux by multiplying the area difference from 2009 to 2019 by the mean ice thickness (Fretwell et al., 2013) at the most inland calving front and the density of ice (ρ=0.9166 Gt km−3). The ice mass change, using a steady-state assumption, was estimated using a flux gate located at the most inland observed calving front position on each ice shelf since 2009, where the mean ice speed, MEaSUREs ice velocity at 450 m resolution, is extracted from the gate location (Mouginot et al., 2019). This is multiplied by the mean ice thickness (Fretwell et al., 2013), the length of the calving front, and the density of ice (Rignot et al., 2013; Table S2). We used the most inland calving front position when calculating ice thickness and velocity to ensure that the fronts were within the spatial coverage of the thickness and velocity datasets. To compare the different methods, we calculated the difference between the two numbers on all ice shelves within the study. We observed mass loss on 18 ice shelves and mass gain on 16. Overall, the steady-state assumption will overestimate ice loss on ice shelves that are advancing and underestimate ice loss on ice shelves that are retreating. The assumption also does not hold well for any irregular behaviour, such as ice shelves that have lost ice through large calving events. Our observations show that Antarctic ice shelves gained 660.6 Gt of ice mass from 2009 to 2019, whereas the steady-state approach would estimate ice loss of −20 028.1 Gt over the same period (Table 1). The steady-state calving flux approximation is closest to the observations on the Antarctic Peninsula; however, the amount of ice loss is significantly overestimated in both West and East Antarctica and on all large ice shelves. These comparisons are in agreement with past studies that compare observed data to steady state (Liu et al., 2015) and show that time-variable observations of calving flux are essential for accurately quantifying the timing and volume of ice shelf calving flux in Antarctica.
Conclusions
This study has generated a comprehensive dataset of change in ice shelf area on 34 Antarctica ice shelves over the last decade. Overall, ice shelves on the Antarctic Peninsula and West Antarctica lost areas of 6693 km2 and 5563 km2, respectively, while East Antarctic ice shelves gained 3532 km2 of ice, and the large ice shelves of Ross, Ronne, and Filchner grew by 14 028 km2 (total). This dataset is a high spatial resolution record of change from 2009 to 2019, which shows the regional differences in ice shelf calving behaviour and documents the frequency and magnitude of ice shelf calving events across the continent on decadal timescales. These observations will be useful for regional studies of ice shelf change in Antarctica and can be used as an input dataset for modelling studies or as a validation dataset for future studies that develop more automated methods of measuring change in ice shelf calving front position. Future studies should use the historical satellite data archives to extend the record of ice shelf area change, which will allow us to establish whether there is a long-term change in ice shelf calving frequency in Antarctica. We must develop and apply automated techniques to increase the frequency with which calving front measurements can be made, particularly on smaller ice shelves and glaciers, which will allow shorter-term, seasonal calving behaviour to be characterised and monitored
Bottom Lines
Mind you, I’m neither a glaciologist nor a data analyst.
That said, I can read, and what I read above—esp. in the methodological parts—is troubling.
Overall, the steady-state assumption will overestimate ice loss on ice shelves that are advancing and underestimate ice loss on ice shelves that are retreating. The assumption also does not hold well for any irregular behaviour, such as ice shelves that have lost ice through large calving events. Our observations show that Antarctic ice shelves gained 660.6 Gt of ice mass from 2009 to 2019, whereas the steady-state approach would estimate ice loss of −20 028.1 Gt over the same period.
The models used until now prognosticated massive ice losses of -20,028.1 Gt from 2009-19—yet, observations show gains of 660.6 Gt during the same period.
Notice, again, the difference between the scientific method (observation) vs. ‘da Science™’ (modelling). While the latter could be said to have been the best of state-of-the-art techniques, empiricism trumps wishful thinking.
Yes, I’m making these bold claims—mainly because the difference between the model vs. the observed realities is only off by a factor of approx. 30.
So, let’s all ‘do our own research’ before blindly trusting ‘da experts™’ on, well, anything.
It is far easier to get the results asked for using models, as the model (just like AI) only shows what it's been fed, which is why models are so popular.
Obvious is obvious, as the kids might say.
Observations are slow, others have access to the same data and can check your work and it's far more difficult to lie using empirical facts.
Models however are also much cheaper. I'd rate models just above free online surveys, when it comes to scientific accuracy.
There is a flaw in trying to use the gains in snow/ice in Antarctica to question global warming patterns. It sounds counter-intuitive, but the rapid and substantial increase in ice is actually predictable and predicated on a warming atmosphere. It's not controversial that warmer air holds more moisture. In Minnesota we would joke that "It's too cold to snow" in January and February, and our heaviest snowfalls were almost always in the late fall or early spring. Air at 20f degrees will hold less moisture than air at 30f degrees, and both will produce snow/ice.